|
|
|
|
Fit of Additional
Data to First Order Creep Model
Kim et al.'s Data and Effect of Cooling
Rate |
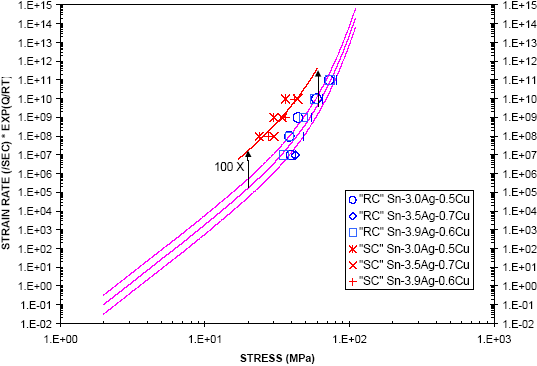 |
Figure
29: Fit of Kim et al.'s data to the first-order SAC
creep model. |
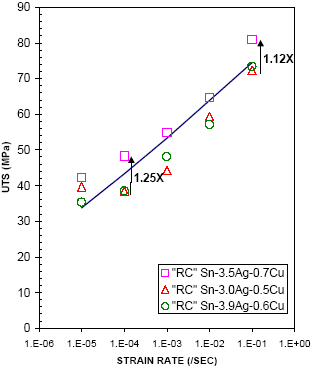 |
Figure 30:
Fit of first-order SAC model (solid line) to Kim et al.'s
data for "Rapidly Cooled" specimens.
Since the first-order SAC creep model given by equation
(38) is essentially empirical, it is important to bounce the
model against additional, independent test data with the goal
of identifying limitations of the model. Figures 29 and 30
show how the model compares to data gathered by Kim et al.
(2001) for Sn-3.5Ag-0.7Cu, Sn-3.0Ag-0.5Cu and Sn-3.9Ag-0.6Cu
tensile specimens. The specimens were either Slowly Cooled
(SC) or Rapidly Cooled (RC) with cooling rates of 0.012°C/sec
and 8.3°C/sec, respectively. The cooling rates were quoted
as average values in the temperature range 230°C to 180°C.
As pointed out by Kim et al., "RC is equivalent to the
cooling speed for soldering in practical conditions in industry".
The raw data, obtained from constant rate stress / strain
tests conducted at room temperature, is given in Table B.3.
The reader is referred to Kim et al.'s paper for further details
on the experimental conditions and the authors' discussion
on creep strength versus alloy composition and microstructure.
Note also that specimens that were Moderately Cooled (MC)
- at a rate of 0.43°C/sec from 230°C to 180°C
- showed similar microstructures and UTS results as for the
RC specimens.
Figures 29 and 30 show that the data for RC specimens fit
the SAC creep model well. In Figure 8, the RC data falls within
or very close to the model correlation band. Figure 30 shows
that the RC data falls on either side of the centerline of
the SAC model (shown as a solid line) and that the effect
of alloy composition is rather small (compared to other effects),
in the range 12% to 25%. The solid line in Figure 30 was obtained
by back-solving equation (39) for stress at a given strain
rate.
On the other hand, Figure 29 shows that the data for the
SC specimens falls outside the correlation band of the SAC
model. The offset in terms of creep rate is a factor of 100
times. This is shown by the solid line going through the SC
data points which is set a factor of 100 times above the centerline
of the SAC model. In terms of stress, and as pointed out by
Kim et al., the strength of SC specimens is about 50% that
of RC specimens.
Clearly, there is a strong effect of cooling conditions
which is explained by Kim et al. in terms of the microstructure.
Cooling rates were not reported quantitatively in the Kariya
et al., Neu et al., Schubert / Wiese et al. publications.
However, Kariya et al. indicated that their specimens were
cooled rapidly ("water-quenched"). Thus, it seems
appropriate that the SAC first order creep model agrees with
the RC data but does not agree with the SC data of Kim et
al.
NCMS Compression Creep Data |
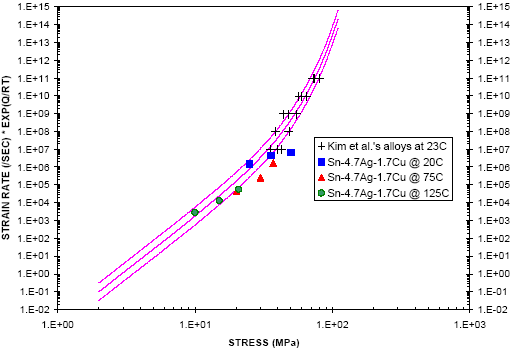 |
Figure 31:
Fit of NCMS Sn-4.7Ag-1.7Cu compression creep data to the first-order
SAC creep model.
Results of creep compression tests at 20°C, 75°C
and 125°C for the NCMS-studied Sn-4.7Ag-1.7Cu alloy (NCMS,
1998) are plotted in Figure 31. Test specimens were short
cylinders of dimensions: 0.4" in diameter by 0.8"
in height. Interestingly, the specimens were cooled rapidly
since, according to the NCMS report, molten solder was poured
into a casting "mold that was chilled". The compression
creep tests were run in load control mode.
The NCMS data falls within or close to the lower bound of
the correlation band of the SAC creep model. Although the
NCMS alloy has higher Ag and Cu contents and the SAC creep
model is based on tensile creep data, the model seems to apply,
at least to a first-order, to compression creep. However,
the fact that the data is slightly to the right of the tensile
master-curve and seems to follow a trendline with different
slopes, suggests that the SAC alloy may also be an uneven
material (similar to Sn-3.5Ag solder). Additional compression
data would be needed to better characterize the compressive
versus tensile response of SAC solders.
The Kim et al.'s creep data for the SAC specimens that were
rapidly cooled is also shown on the same plot (Figure 31)
to illustrate that the two datasets provide for first-order
validation of the creep model at different stress levels:
above 35 MPa for the Kim et al.'s "RC" data, below
35-50 MPa for the NCMS data.
Flip Chip Solder Joint Shear Data |
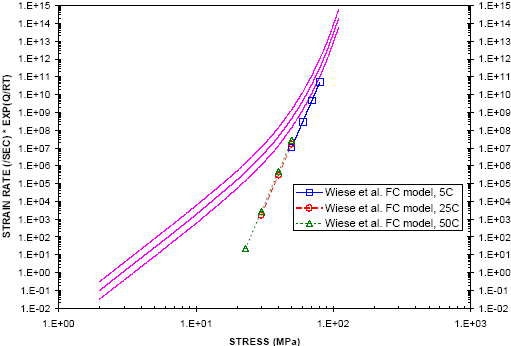 |
Figure
32: Flip-chip solder joint shear model versus master-curve
of bulk SAC tensile creep model.
Wiese et al., 2001, tested flip-chip solder joints of composition
Sn-3.8Ag-0.7Cu in shear at 5°C, 25°C and 50°C.
Their data (shown in Figure 20 and 23 in Wiese et al., 2001)
was fit to the following power-law model: |
 |
(40) |
The above model is plotted on Figure
32 as: |
 |
(41) |
where Q = 67.9 kJ/mole is the
activation energy of the SAC creep model. Data points that
are shown for equation (41) are calculated at temperatures
of 5°C, 50°C and 50°C and in the stress range
of the original test data obtained by Wiese et al.
Clearly, the flip-chip creep model and the master-curve of
the tensile SAC creep model follow different trends. A similar
difference between creep in shear and in tension was pointed
out by Wiese et al., 2001. There are several possible reasons
for this, although it is not clear how these differences can
be resolved:
- Significant differences in micro-structures and dispersion
of chip metallization elements or
intermetallics in the flip-chip joints, the effects of which
are not captured in the SAC creep model.
- Equation (40) was obtained by fitting shear creep data
that were converted to tensile data using the uniaxial stress
and strain transformations:
=  3
and
= / 3.
The latter are based on the application of a multi-axial
Von-Mises yield criterion. This widely-used criterion is
for materials with time-independent plastic flow and, in
general, has not been verified under creep conditions. The
applicability of the Von-Mises criterion to SAC solders
has not been demonstrated either and may be questionable
as in the case of Sn3.5Ag.
- The shear creep data derived by Wiese et al. converts
shear forces and displacement rates into average shear stresses
and shear strain rates assuming a uniform shear distribution
in the minimum section of hour-glass shaped flip-chip joints.
While this is a first-order engineering approach at handling
the force-displacement rate data, it may be an over-simplifying
assumption because of the complexity of shear strain distributions
in solder joints.
A similar discrepancy between creep in shear and in tension
has been reported by Darveaux et al., 1995, for Sn-Pb and
Sn-3.5Ag solders. |
|