Bulk Sn-3.5Ag Tensile Creep
Source and Plot
of Data
|
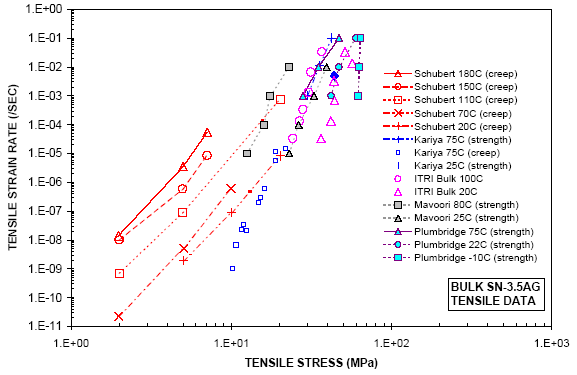 |
Figure 8: Log-log plot of isothermal tensile
creep data for bulk Sn-3.5Ag solder.
Isothermal tensile creep data from FIVE independent
sources are plotted as strain rate (/second) versus tensile
stress (MPa) in Figure 8. The data covers about two orders
of magnitude on the stress axis (from 2 to 64 MPa) and ten
orders of magnitude on the strain rate axis (from 2.2 x
10-11 to 10-1 /sec). Test temperatures are in the range
-10°C to 180°C. The raw data (59 points total) is
listed in Table A.1 in Appendix A. The data was obtained
from the following figures and tables in their respective
publications:
|
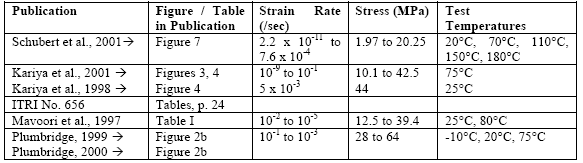 |
Most tests were tensile "strength"
tests carried out at a constant strain rate. Schubert et al.
conducted constant load creep tests. Kariya et al. ran both
constant load and constant strain rate tests. Note that only
the Schubert data covers the low stress region below 10 MPa.
This is the region of most interest for solder joints of electronic
assemblies under a wide range of use conditions. |
Specimens |
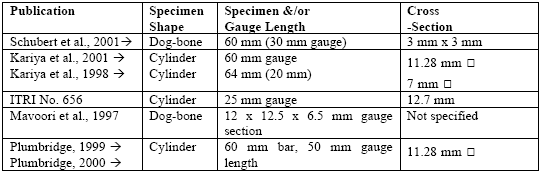 |
Table 6:
Bulk Sn-3.5%Ag tensile specimen geometries. |
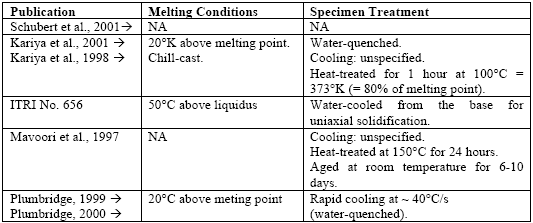 |
Table 7: Bulk Sn-3.5%Ag tensile specimen
treatments (NA = not available).
Test specimens were dog-bone or cylinder-shaped
tensile specimens with specimen and/or gauge length and
cross-sectional dimensions given in Table 6. Melting and
cooling conditions as well as thermal treatment of specimens,
when available, are summarized in Table 7. The reader is
referred to the original publications for additional details
on specimen preparation. Comparing the specimen information
from the five sources, note that:
|
|
- The above dimensions compare to typical sizes of 0.1 to
0.2 mm (4 to 8 mils) for flip-chip solder joints or 0.5
mm (20 mils) for Ball Grid Array (BGA) solder joints. That
is, specimen dimensions are one to two orders of magnitude
larger than dimensions of actual solder joints.
|
- The Kariya et al. specimens were rapidly cooled by water-quenching
while cooling conditions for
others were not reported. Some specimens were heat-treated
while no heat-treatment is reported for others. Heat-treatment
conditions also differ among laboratories, e.g. 24 hours
at 150°C (Mavoori et al.) versus 1 hour at 100°C
(Kariya et al.).
|
Microstructure |
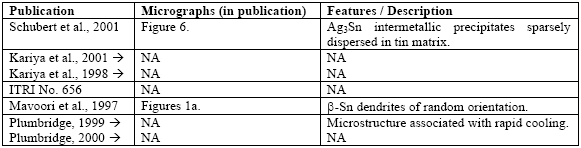 |
Table 8:
Microstructure of bulk Sn-3.5Ag tensile specimens (prior to
testing).
The microstructure of the as-cast Sn-3.5Ag solder consists
of a b-Sn matrix with dispersed Ag3Sn intermetallic phases.
Specific microstructural features for the alloys and specimens
under study are given in Table 8, when available. |
Data Analysis |
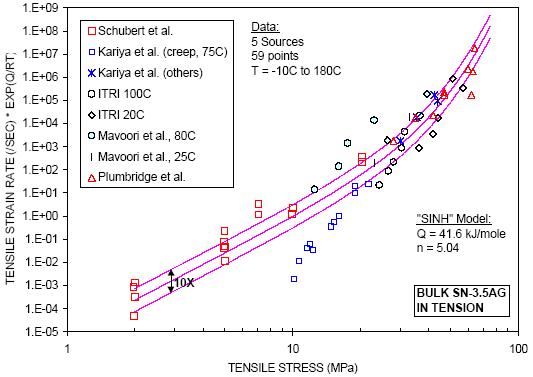 |
Figure 9: Curve-fitting of bulk Sn-3.5Ag
tensile creep data to hyperbolic sine model.
Centerline is a plot of
versus
where
is given by equation (28).
In an attempt to consolidate the data in Figure
8, the creep rates are fitted to a hyperbolic sine model:
|
 |
(26) |
which gives the calculated steady-state
strain rate:
(/second) as a function of stress (MPa)
and absolute temperature T (°K).
special definitions: |
|
R |
universal gas constant, 8.314 J/(°K.mole)
~ 2 cal/(°K.mole). |
|
|
A, B, n |
model constants |
|
|
Q |
activation energy |
|
A, B, n, and Q are obtained by regression of the data in
Table A.1. The regression was done using the non-linear, multiple
variable curve-fitting program "Datafit" by Oakdale
Engineering (http://www.curvefitting.com).
Because of the wide range of low strain rates, the
analysis was conducted on
and the regression function was specified as: |
 |
(27) |
where T(°K) and
(MPa) are entered as independent variables and the regression
constants LNA and Qa are defined as: LNA = ln(A)
and Qa = Q/R. The regression results are given
as central values with standard deviations:
- LNA = 3.1429 ±3.427 (from which the central value
of A is: A = 23.17)
- B = 0.0509 ± 0.0125
- n = 5.04 ± 0.64
- Qa= 5000 ± 1086 (from which the central
value of Q is: Q = 41.57 kJ/mole ~ 0.43 eV)
Note that the constant LNA has the largest standard deviation
error and the error on the activation energy is ±22%.
That is, the range of activation energies is: 32.5 to 50.6
kJ/mole. The equation of the centerline in Figure 9 is: |
 |
(28) |
The dashed lines in Figure 9 are an arbitrary
factor 10
= 3.16 times above and below the best-fit line.
Discussion
The spread of the data around the centerline in Figure 9 highlights
the difficulties encountered when trying to consolidate data
from multiple sources or when using a first order creep model
such as the hyperbolic sine model. Often, these discrepancies
are not shown since many publications do not include comparisons
of raw data to independent test results. We conclude that:
- Simplified creep models such as the one in equation (26)
or other models that are fit to data from a single source
should be used with caution. In the latter case, creep models
may not be as encompassing as it appears and these models
should be bounced against data from independent experiments.
- Much work remains to be done to resolve possible discrepancies
among the Sn-3.5Ag tensile datasets and before a reliable
constitutive model can be developed for small solder joints
of electronic assemblies.
- Similar challenges hold for other lead-free solder alloys.
Comparison to Sn-4Ag Tensile
Creep Data |
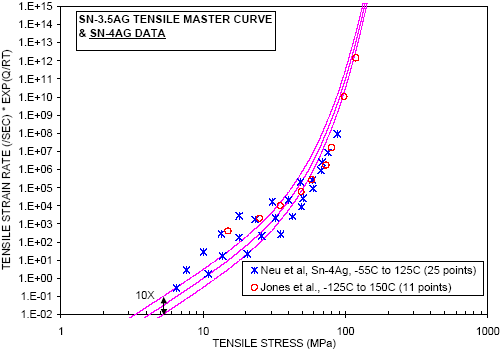 |
Figure
10: Sn-4Ag tensile creep data compared to master
curve of Sn-3.5Ag tensile creep data.
Figure 10 shows Sn-4Ag creep data on the same plot as the
Sn-3.5Ag tensile master curve from Figure 9. The raw data,
digitized from Figure 3 in Jones et al. (1998) and Figure
10 in Neu et al. (2001), is listed in Table A.2. Basic specimen
information was given as follows:
- For the Jones et al. experiment: cast, water-chilled
tensile specimens, 40 mm long (15 mm gauge length), 2 mm
thick. Isothermal tensile tests were strain-controlled.
- For the Neu et al. data: cylinders 127 mm long by 12 mm
in diameter (alloy was heated 50°C above liquidus, mold
was air-cooled), gauge section machined to 15.3 mm in length
and 6.35 mm in diameter. The data is from isothermal strain-rate
jump tests.
Sn-4Ag, with a melting range from 221°C to 228°C,
has a composition near that of the eutectic Sn-3.5Ag alloy.
Although the Sn-3.5Ag master curve is an approximate fit through
the Sn-3.5Ag data, Figure 10 shows that, to a first order,
the Sn-4Ag data points are reasonably close to the correlation
band for Sn- 3.5Ag. That is, the creep resistance and tensile
strength of two alloys with rather close silver contents (4%
vs. 3.5%) appear to follow similar trends. |